Experiments
Experiments
We are pursuing a number of experiments in quantum information and with a particular emphasis on macroscopic quantum effects. This includes spectroscopy of single organic molecules and using single photons to entangle organic molecules.
We are developing efficient optical and molecular switches for quantum computation as well as optical waveguides in order to simulate complex quantum systems. We are particularly interested in the universal features of quantum physics: do genuine quantum effects exist in living systems and can they be relevant at the cosmological scales?
Overview – quantum thermodynamics in practice
The objective is to develop optical transistors integrated in digital quantum simulators. Optical transistors use light instead of electrical current to switch between 0 and 1 bits and offer advantages as to low power consumption and faster bit-flip rates. They could help palliate the rapid growth in requirements for bandwidth and energy-efficiency due to data storage and analysis. Optical transistors have become possible thanks to the convergence of two key enabling technologies, (i) photon sources based on organic dye molecules, and (ii) photonic integrated circuits (PICs). The engineering challenge is to scale-up the transistor into a network and to optimise the signal-to-noise ratio.
Underpinning this effort are deeper questions as to the exact formulation of quantum thermodynamics, a new branch of quantum physics and engineering that treats microscopic systems (at the level of atoms and single molecules) as quantum heat engines. A better understanding of their behaviour will revolutionise computing by reducing the energy per computing step, ultimately leading to frictionless computers that violate the laws of classical thermodynamics, hitherto believed to be unimpeachable.
Our two experimental deliverables are detailed here.
(i) Optical transistor based on photon sources from organic dye molecules – We use dibenzoterrylene molecules (DBT) as an ideal sources of indistinguishable (quantum) photons. Unlike any other photon source to date, DBTs are cheap and emit almost perfectly reproducible lifetime- limited spectral lines generating uni-polarised single-photons from a two-level transition at 785nm, a technologically important wavelength because it matches the transmission window of telecom fibres. As such they offer an ideal source of qubits for applications in quantum information processing. Moreover, proof-of-principle experiments have already demonstrated that it is possible to integrate DBTs into silicon-based photonic light circuits (PLCs), ubiquitous in the telecommunications industry, and to configure them into optical transistors. Here we fabricate compact optical transitors based on DBTs with a view to integrating them into PLCs. The objective is to optimise their design and performance into patentable photonic devices. Beyond optical transistors, along the way it will be possible to spin off devices that generate entangled-qubits, which can be plugged into distributed quantum networks, or as components for quantum random number generators. Devices of this kind that can demonstrate reliable performance are in very high demand in the emerging field of quantum technologies, and all benefit from low- power consumption and compactness inherent in integrating photon sources directly with waveguides. (ii) Digital quantum simulators (in Silicon Photonic Light Circuits and Photonic Integrated Circuits). Here we use silicon-based Photonic Light Circuits as a platform technology to implement
quantum optical digital simulators and integrate optical transistors. Digital quantum simulators offer a cost-effective tool to simulate the behaviour of quantum systems. The industrial appeal of quantum simulators is apparent when we consider that such circuits can encode the quantum behaviour of say pharmacological reagents at the microscopic level, or within the context of artificial intelligence, a machine learning protocol.

Figure: Blueprint for a photonic light circuit currently in use in our laboratory. Under the action of projective (quantum) measurements performed on the middle potential well the dynamical evolution of the system is frozen (Zeno effect) when the interval between consecutive measurements is small compared to the tunnelling rate.
Our objective here is to design, fabricate and pre-programme a digital quantum simulator, and to make it mimic the behaviour of a quantum system, say an ion in potential wells and others. As a proof-of-principle demonstration it will showcase the ability of such devices to obviate the need for costly experiments. The simulation of the evolution of an ion trapped in a lattice of three coupled potential-wells across which it undergoes tunnelling is a scenario that draws wide interest, since it is one route towards quantum information processing.
Our digital simulators encode this behaviour in an architecture comprising up to 100 cascaded Mach Zehnder interferometers that reconstruct the full physical results of the system. A natural extension of this objective will be to make the PICs programmable, which entails
integrating optical transistors and switchable phase shifters in a bid to realise the world’s first universal optical quantum processor.
A selection of our current topics of interest are outlined below:
Single photon probes
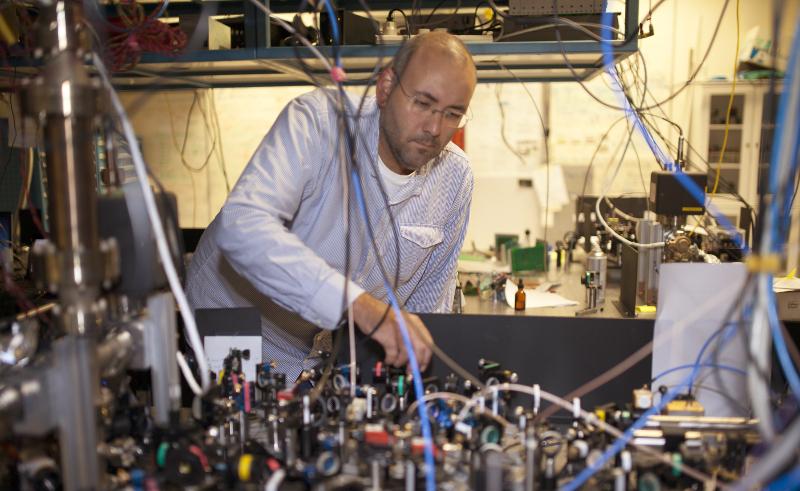
Quantum biology
